Developing point-of-care CAR T manufacturing
Posted: 27 December 2023 | Professor Arnon Nagler (Tel Aviv University) | No comments yet
Driven by technological advances there is now increased scope for point-of-care manufacturing of CAR T-cell therapy. Arnon Nagler, Professor of Medicine at Tel Aviv University, discusses key benefits, challenges and lessons learned from developing an in-house programme.
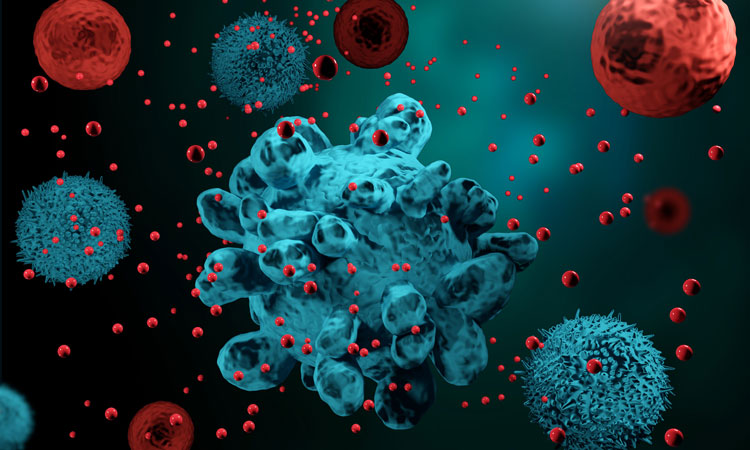
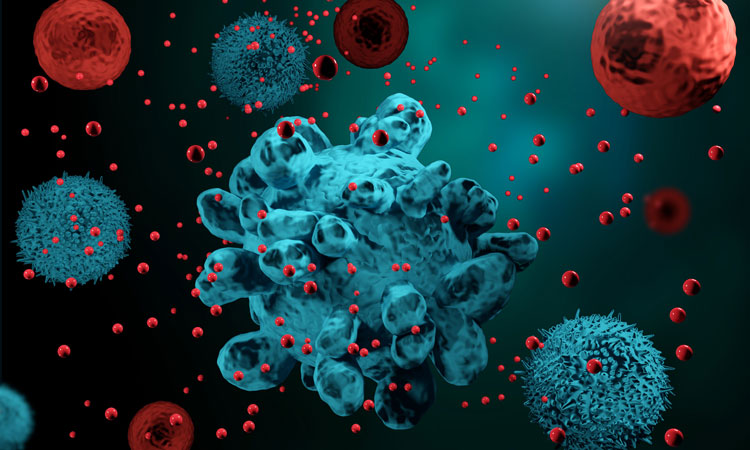
Chimeric antigen receptor (CAR) T cells are the first example of a “living drug”.1 Following in vitro production from T cells collected from a patient’s leukapheresis procedure, the CAR T cells are infused back into the patient’s blood, where they proliferate and expand. The CAR T cells need to persist in the blood to expand and elicit maximal effect, and there is a correlation between expansion and persistence and response.
Currently CAR T-cell therapy is approved for three indications: acute lymphoblastic leukaemia (ALL), non-hodgkin lymphoma (NHL), and multiple myeloma (MM) – and the strongest correlation between CAR T-cell efficacy and persistence has been demonstrated in ALL.2,3
Clinical implementation of CAR T-cell technology requires a reproducible T-cell manufacturing platform, which necessitates effective gene-transfer tools and T-cell culture conditions. Being a cellular therapy that involves cell manipulation and molecular transfection – and above all, being a living drug – makes CAR T-cell therapy a somewhat challenging immunotherapy to manufacture in‑house.
Considerations for in-house CAR facilities
When establishing a point-of-care CAR facility, there are several initial considerations that must be addressed. First, leukapheresis – the first step in producing CAR T cells – is usually carried out in transplant departments or processing facilities. These facilities are experienced in haematopoietic stem cell mobilisation, harvesting for haematopoietic stem cell transplantation, as well as leukapheresis for donor lymphocyte infusions. Second, the production and delivery of CAR T cells necessitates close collaboration between the pharma industry and academic (usually transplant) departments. In other words, the apheresis centres must become an integral part of the pharmaceutical production line with identical quality assurance and regulations, which is new and unpreceded for both sides.
production and delivery of CAR T cells necessitates close collaboration between the pharma industry and academic departments
CAR T production slots are assigned based on availability and a periodic quota system per referring institution or geographic location. Product distribution requires shipping of apheresis starter materials, either cryopreserved or fresh (depending on the product), to the manufacturing site as well as shipping of final cryopreserved products to treatment centres. This round-trip shipping was especially challenging during the early days of the COVID-19 pandemic, which coincided with initial attempts to establish CAR T-cell therapies in daily practice. Due to shipping and manufacturing requirements, the time from a patient being included in the CAR T programme to receiving therapy can be several weeks. This is variable and to some extent unpredictable. As a result, many patients waiting for the CAR T cells to be infused will need additional therapy (bridging therapy) against the malignance. Bridging therapy has been shown to correlate with an inferior response to CAR T-cell therapy due to both progression of the chemotherapy-refractory malignant disease during the manufacturing period and increased toxicity.4
In addition, multi-disciplinary teams must be established for in-house CAR T-cell therapy programmes to ensure maximum success and safety. The first patient ever to receive CAR T-cell treatment (a child with ALL) developed severe life-threatening cytokine release syndrome (CRS) early post CAR T-cell administration and needed intensive care unit (ICU) intervention.5 Subsequently, transplant centres administering CAR T cells in various pharma-sponsored clinical trials had to establish multi-disciplinary teams, including not just haematologists and oncologists but also intensive-care physicians and nurses, nephrologists, neurologists, pharmacists and others. This is especially important due to the two unique toxicities of CAR T cells, namely CRS and the neurology toxicities that subsequently received the term immune effector cell- associated neurotoxicity syndrome (ICANS). After this incident CAR-related toxicity scales and guidelines were established to prevent adverse reactions.
Lessons learned
The process of establishing point-of-care CAR T manufacturing was a learning curve, not just in managing the toxicities and side effects, but also in understanding the important difference between emerging CAR T-cell adoptive immunotherapy and traditional stem cell transplantation. Since CAR T-cell clinical development and administration was partially based on the knowhow and experience gained in the stem cell transplantation field, it is important to understand and recognise the differences between the two medical cellular therapies, including the indications they treat and their patient workups.
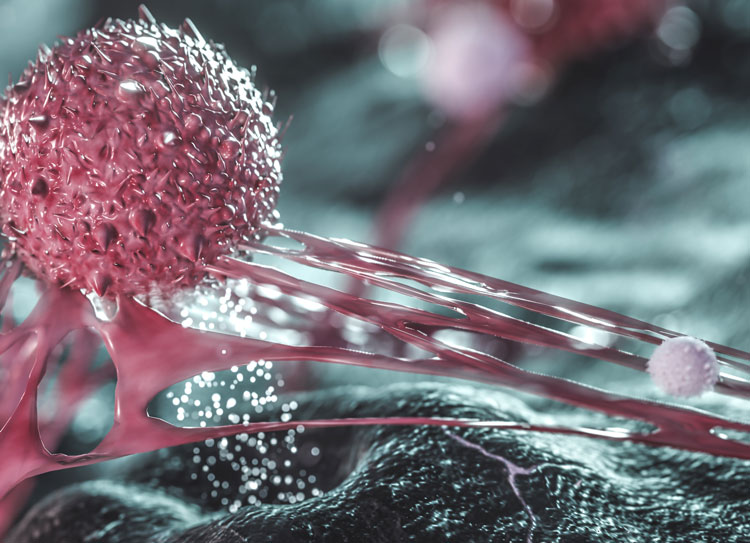
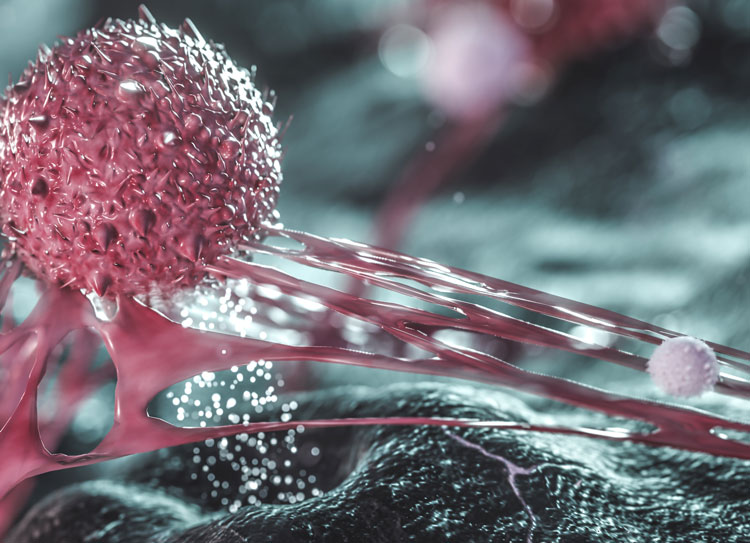
Building on success with haematological malignancies, CAR T-cell therapy holds promise for treating solid tumours.
Building on success with haematological malignancies, CAR T-cell therapy holds promise for treating solid tumours.
The most important difference between CAR and stem cell transplantation is their contraindications. For example, central nervous system (CNS) involvement is a contraindication for stem cell transplantation; it was therefore initially a contraindication for CAR T-cell therapy in pharma‑based registry studies, but this is no longer the case. Other examples include the absence of age requirement/limitation for CAR T-cell therapy and that chemo sensitivity is not mandatory for CAR treatment.6-8
Clinical recommendations also change. For example, the use of granulocyte colony stimulating factor (G-CSF) in cases of early severe or prolonged haematological toxicities was not allowed initially because of immunological properties. Additionally, it was not recommended to use low-dose steroids or allow early administration of tocilizumab, a monoclonal antibody targeting the interleukin-6 (IL-6), in order to reduce and prevent severe CRS. Studies have since demonstrated significantly reduced CAR-associated CRS and other toxicities without jeopardising therapeutic yield and outcomes;9 both G-CSF and tocilizumab are now part of daily practice in CART-cell therapy.
Important reassurance about CAR T-cell therapy came with the real-world data that emerged from both large single centres and various consortiums, which included data from both adult and paediatric patients in Israel and Europe. This data has been captured by CAR T-cell registries that were established first by the Center for International Blood and Marrow Transplant Research (CIBMTR) and later by the European Society for Blood and Marrow Transplantation (EBMT).10
Benefits of point-of-care CAR T-cell therapy
Even after validation of the efficacy and safety of the therapy, two critical challenges remained – affordability and accessibility. The demand for commercial CAR products is very high due to its unprecedented success in treatment of patients with very advanced haematological malignancies. Patients seeking CAR T-cell therapy have already tried many lines of therapy and exhausted other available treatment options; CAR T-cell therapy is for the first time providing hope to these patients. The demand for CAR T-cell therapy is therefore much greater than the supply, making it difficult for patients to access the potentially life-saving treatment. This is in addition to its considerable price tag.
Manufacturing and administering CAR T-cell therapy close to academic centres and treating haematologists, oncologists and researchers has led to important scientific discoveries
Manufacturing CAR T cells in-house, however, is increasing accessibility of the treatment for patients, and drastically reducing associated costs. Furthermore, point-of-care CAR T-cell manufacturing provides clinical benefits. By keeping the process entirely in-house, the vein (patient leukapheresis) to vein (CAR T-cell administration) time is reduced to about 10 days, which spares patients the necessity of bridging therapy, correlating with improved outcomes.
The in-house production of CAR T-cells additionally allows attending physicians to tend to urgent clinical demands or acute changes in a patient’s clinical status. On the logistics side, the in-house process eliminates, in principle, the need for cryopreservation of the CAR T-cell products. The administration of non-cryopreserved products may also reduce cell and potency loss.
CAR administration leads to further industry advances
Manufacturing and administering CAR T-cell therapy close to academic centres and treating haematologists, oncologists and researchers has led to important scientific discoveries. Examples include discovering the importance of T-cell fleetness and stemness for the potency of the CAR T-cell products, as well as new and novel CAR constructs with better expansion capabilities and persistence.11 These initial findings were further developed by industry; in many instances pharma companies provided funding while working hand-in-hand with academia to advance the field, benefitting patients. In parallel, the industry developed automated/fast producing systems, further improving accessibility of CAR T-cell therapy.
The cost of in-house manufacturing
In-house point-of-care CAR T-cell programmes come at a cost and require a major effort to establish.
Developing an in-house CAR T-cell programme necessitates current good manufacturing practice (cGMP) facilities, trained and capable personnel (production and quality control), equipment and knowhow, and of course budget to ensure product quality, to meet complex regulatory requirements and receive the mandatory approvals. These criteria may put constraints on smaller facilities and make it unfeasible. However, recently developed automated close systems make in-house production of CAR T cells and CAR T-cell academic programmes a realistic possibility for an increasing number of centres.
The CAR T field will gain from continued close collaboration between academia and industry, and the rapid development of new CARs best exemplifies the importance of this joint work. CAR T cells revolutionised modern haematological malignancies treatments, curing patients that until recently had no hope. Many more CAR developments are on the horizon and the field is awaiting similar breakthroughs for treatment of solid tumours, which are currently in early stages of development.
About the author
Professor Arnon Nagler, MD is President of the Hemato-Oncology Center and director of the Division of Hematology and the Bone Marrow Transplantation and Cord Blood Bank at the Sheba Medical Center, and Professor of Medicine at Tel Aviv University in Israel. Professor Nagler has been working in the field of bone marrow transplantation for haematological malignancies for the last 25 years. He established the first public cord blood bank in Israel and performed the first cord blood transplantations from related and unrelated donors in genetic and malignant haematological diseases. Prof Nagler received his medical training at the Hebrew University-Hadassah Medical School, Jerusalem, specialising in Internal Medicine and Hematology at the Rambam Medical Center, Haifa, and in Hematopoiesis at TA University.
References
- Liu D. Car-t “The living drugs”, immune checkpoint inhibitors, and precision medicine: A new era of cancer therapy. Journal of Hematology & Oncology. 2019;12(1).
- Sengsayadeth S, Savani BN, Oluwole O, Dholaria B. Overview of approved car‐t therapies, ongoing clinical trials, and its impact on clinical practice. eJHaem. 2021;3(S1):6–10.
- Center for Biologics Evaluation and Research. Approved cellular and gene therapy products [Internet]. US Food and Drug Administration. FDA; 2022 [cited 2023 Mar]. Available from: https://www.fda.gov/vaccines-blood-biologics/cellular-gene-therapy-products/approved-cellular-and-gene-therapy-products
- Sun Z, Liu MS. Systematic Review and meta-analysis of the association between bridging therapy and outcomes of chimeric antigen receptor T cell therapy in patients with large B cell lymphoma. Cytotherapy. 2022;24(9):940–53.
- Grupp SA, Kalos M, Barrett D, et al. Chimeric antigen receptor–modified T cells for acute lymphoid leukemia. New England Journal of Medicine. 2013;368(16):1509–18.
- Mirza A-S, Hosing C, Foss FM, et al. Impact of age on outcomes after CD19 directed car T cell therapy for large B cell lymphomas: Real world experience from the Center for International Blood & Marrow Transplant Research (CIBMTR). Blood. 2022;140(Supplement 1):4633–5.
- Zhang H, Liu M, Li Q, et al. Evaluation of the safety and efficacy of humanized anti-CD19 chimeric antigen receptor T-cell therapy in older patients with relapsed/refractory diffuse large B-cell lymphoma based on the Comprehensive Geriatric Assessment System. Leukemia & Lymphoma. 2021;63(2):353–61.
- Asghar N, et al. Chimeric antigen receptor T-cell (CAR T-cell) therapy for primary and secondary central nervous system lymphoma: A systematic review of literature. Clinical Lymphoma Myeloma and Leukemia. 2023;23(1):15–21.
- Rejeski K, Perez A, Sesques P, et al. Car-HEMATOTOX: A model for car T-cell–related hematologic toxicity in relapsed/refractory large B-cell lymphoma. Blood. 2021;138(24):2499–513.
- Schultz LM, Baggott C, Prabhu S, et al. Disease burden affects outcomes in pediatric and young adult B-cell lymphoblastic leukemia after commercial tisagenlecleucel: A pediatric real-world chimeric Antigen Receptor Consortium Report. Journal of Clinical Oncology. 2022;40(9):945–55.
- Hayden PJ et al. Management of adults and children receiving car T-Cell therapy: 2021 best practice recommendations of the European Society for Blood and Marrow Transplantation and the Joint Accreditation Committee of ISCT and EBMT and the European Haematology Association. Annals of Oncology. 2022;33(3):259–75.
Issue
Related topics
Clinical Development, Drug Manufacturing, Immunotherapy, Manufacturing, Personalised medicine, t-cells
Related organisations
Related diseases & conditions
Acute lymphoblastic leukaemia (ALL), Cytokine release syndrome (CRS), multiple myeloma (MM), non-Hodgkin lymphoma (NHL).